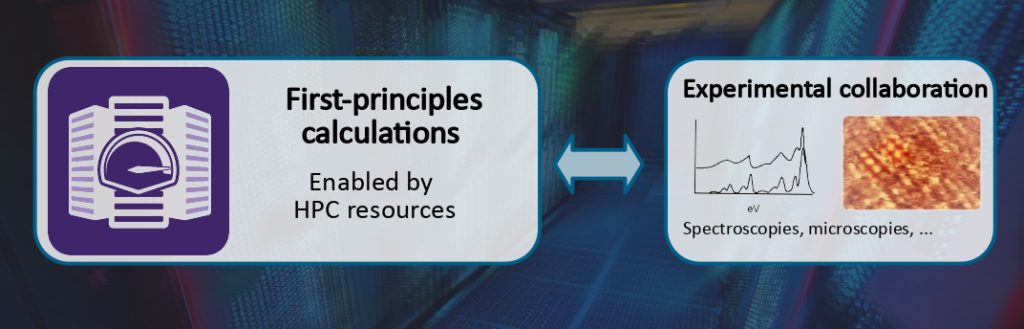
We seek to describe and engineering how defects and interfaces impact the atomic and electronic structure of extended semiconducting materials relevant to energy sustainability technologies.
We use methods based on density functional theory, molecular dynamics, and many-body perturbation theory to achieve a microscopic understanding of the electronic, optical, and transport properties.
Electrocatalysis for
water-splitting reactions
Hydrogen fuel is considered to be a promising candidate to replace fossil fuels. Water splitting via electrolysis is a promising avenue for generating hydrogen fuel in an efficient manner. We are interested in complex oxides and the transition metal oxyhydroxides as electrocatalyst platforms.
Our goal is to develop design principles that can enhance activity and stability of electrocatalysts by computational simulation the electrode material and electrode/aqueous interface.
Photocatalysis for
hydrogen production
Harnessing solar energy to drive water splitting reactions is a holy grail to sustainable and clean generation of hydrogen. A critical component is simultaneously optimizing the optical properties, electronic transport, and surface chemistry of the photocatalyst.
Our goal is to understand how structural heterogeneities in layered materials, such as defects and layering effects, impact and can be used to alter the photocatalytic behavior.
Designer defects for next-generation computing technologies
New materials are needed to meet the projected demands of computation and memory. Computing devices beyond the von Neumann architecture will be necessary to achieve energy-efficient computing. One example is neuromorphic computing, as inspired by the energy-efficient computations performed by the human brain. We are developing materials design criteria of defects in semiconductors to predict the electrical properties and characteristics of ultra-thin materials using a combination of electronic structure and methods inspired by machine-learning techniques.